Society: AGA
Introduction
Gastric motility is coordinated, in part, by rhythmic bioelectrical events called slow waves. Dysrhythmic slow waves with abnormal propagation patterns have been associated with a number of motility disorders, including functional dyspepsia and gastroparesis, which affect approximately 10% of the global population.
High-resolution mapping of slow waves has emerged as a promising diagnostic tool for classifying gastric electrical dysrhythmias, however, current methods for generating detailed maps of gastric electrical activity to guide targeted therapy are surgically invasive. This study aimed to validate the clinical application of a novel, minimally-invasive, endoscopic device for high-resolution mapping in humans.
Methods
A novel endoscopic mapping device was constructed that primarily comprised of a Constellation cardiac mapping catheter (60 mm diameter, 8 splines, 64 electrodes; Boston Scientific, USA), and anorectal balloon (Mui Scientific, Canada) positioned inside the mapping catheter splines (Fig. 1A).
Ethical approval and informed consent (HDEC approval #17/STH/95) was gathered from patients undergoing elective gastroscopy to participate in the study. Patients were placed in the prone position prior to device deployment to minimize potential movement. The device was then advanced into the gastric antrum, alongside a gastroscope, using an endoscopic clip to hold the tip of the device. The balloon was inflated and the stomach suctioned to maintain contact between the mucosa and electrodes (Fig. 1C). Electrical recordings were obtained for approximately 7 min, following which the device was deflated and removed. Signals measured by the novel mapping device were digitally recorded by a Biosemi ActiveTwo system (Biosemi, Amsterdam, Netherlands) at a sampling frequency of 512 Hz, prior to local storage. Data were then analyzed in custom software to detect and quantify slow wave activation patterns.
Results
Device placement and data acquisition was successful in all patients (n=13). Slow waves were recorded with frequency 2.8±0.5 cycles/min, velocity 4.3±1.4 mm/s, and amplitude 2.6±1.9 mV. These values are within an established physiological range of antral slow wave propagation, demonstrating validity of our new endoscopic device. Slow wave detection was achieved with sufficient channel coverage (>33%), allowing spatiotemporal propagation map generation (Fig. 2B), in 5 of the patients.
Conclusions
This study demonstrates the first endoscopic, minimally-invasive, high-resolution slow wave mapping in humans, using a novel device design. This device could be used to localize gastric dysrhythmias in the future as a diagnostic tool, and to guide emerging treatments. Future studies will expand the patient cohort to compare gastric slow wave activity in healthy and diseased patients.
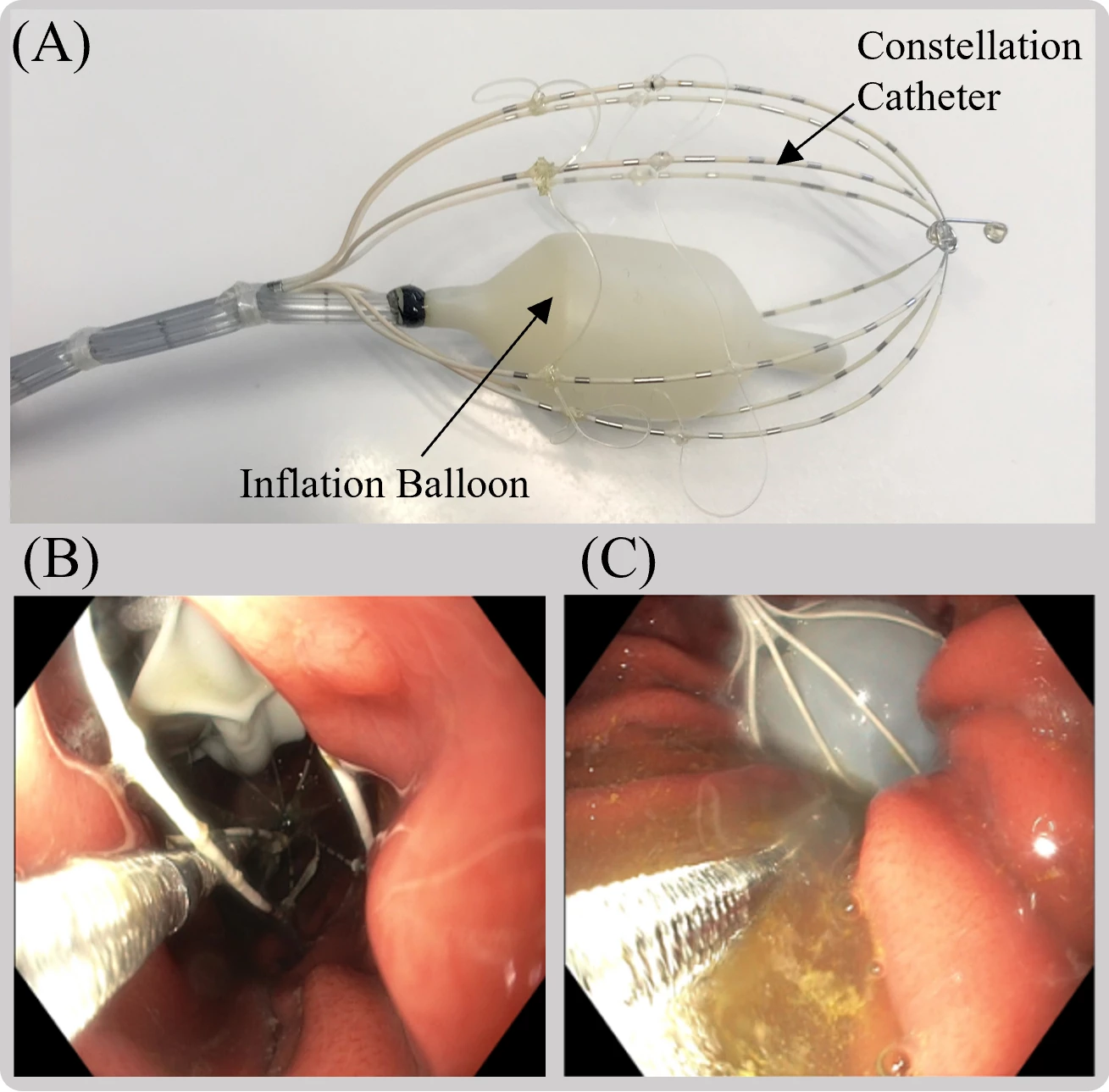
Figure 1: (A) Recording tip of novel endoscopic device with primary components labelled (B) Device positioned in distal antrum of patient prior to inflation (C) Device inflated and ready to begin recording.
Figure 2: (A) Representative electrogram from patient. Slow wave activation points are marked with red dots. The wave group highlighted in the blue band of electrogram (A), is visualized in the propagation map presented in (B). Isochrones are stepped at 0.5s intervals for propagation maps. Direction of slow wave propagation indicated with black arrow.
Introduction: While colonoscopy is the gold standard for diagnosing colorectal adenomas, it has limitations, including the use of conscious sedation and missed polyps frequently reside behind colonic folds. To overcome these limitations, we have developed a Retrograde Tethered Capsule Endomicroscopy (R-TCE) technology that is designed to image the entire colon at the microscopic level without requiring patient sedation. Here we describe our initial experience in living swine with this new technology.
Materials and Methods: The R-TCE device is a 11x25 mm capsule with silicone external threads attached to a 2-meter-long, ~ 4-mm-diameter, flexible tether. The tether contains a drive cable that rotates the capsule, an external 22-mm-diameter inflatable balloon at its distal tip, and a second channel for water delivery, as shown in Figure 1. After the capsule is inserted into the anus, rotation of the capsule at 15 rpm propels it up the large intestine via a screw-like motion. The capsule also implements optical coherence tomography (OCT) with integrated micromotor scanning (20 Hz) obtains 20 µm resolution cross-sectional images of the entire colonic wall.
R-TCE device prototypes were tested in n=5 Yorkshire swine (w=50 kg) in vivo. Bowel-prepped animals were anesthetized before the experimental procedure. The capsule was inserted in the anus, rotated, and progressed up the swine’s spiral colon approximately 1 m. Once the capsule reached its terminal location, the swine underwent multi-angle X-ray abdominal fluoroscopy to record the tether’s anatomical shape and position. Then, the balloon was inflated and the capsule pulled back at a rate of 1 mm/s, acquiring helical 3D microscopic OCT images of the entire large intestine. To evaluate whether R-TCE produced any mucosal damage, a gastroenterologist conducted a colonoscopy in the swine. After animals were sacrificed, histology was taken from regions of the colon with visible damage caused by either the R-TCE device or the colonoscope. A pathologist scored these slides using a histopathologic mucosal damage scoring system (R-TCE vs. colonoscopy)
Results: R-TCE enabled full circumferential visualization (95.94±0.13%) of the colon of ~1 m in vivo (n=5). As shown in Figure 2, 3D fly-throughs rendered from OCT images showed excellent visualization of the colon’s folds. The R-TCE histopathology mucosal damage score (8±2.6) was statistically equivalent to that of colonoscopy (9.5±4)(p =0.19, n=3).
Conclusion: We have successfully demonstrated a new tethered capsule device in living swine that obtains 3D microscopic OCT images of the entire colon. Preclinical safety is supported by statistically equivalent superficial mucosal damage caused by R-TCE and colonoscopy. Future work will involve first-in-human studies to evaluate the tolerability and imaging capabilities of this new technology in unsedated human subjects.
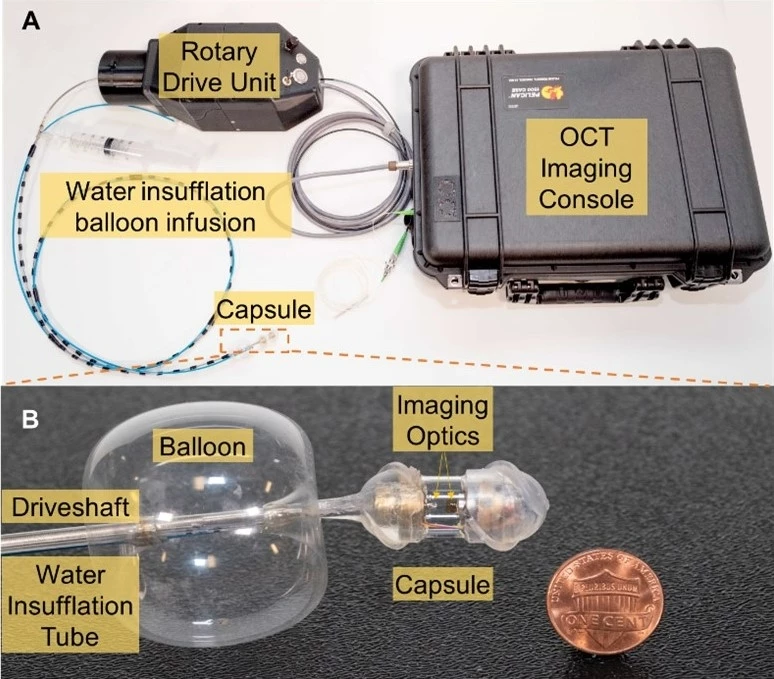
Figure 1. Photos of the R-TCE device. A. R-TCE portable OCT imaging console, capsule rotational drive unit, water insufflation channel, and capsule B. Magnified view of the R-TCE capsule, inflated balloon, and tether interface.
Figure 2. A. 3D reconstruction of the colonic lumen derived from OCT images acquired by the R-TCE device and multi-angle abdominal x-rays in vivo. Linea alba and multiple haustra can be seen. B-D. Representative circumferential, cross-sectional OCT images of the spiral colon, transverse colon, and rectum, respectively. E. 3D rendered fly-through view of OCT data, obtained from the colon in vivo, showing multiple colonic folds. # indicates the micromotor wire, + an area where the capsule was in contact with mucosa, and * an area where the colon wall was outside of the OCT imaging range. Labels 1,2,3 indicate colonic folds.
Background Fecal calprotectin (FCP) is a non-invasive surrogate marker of mucosal inflammation frequently used to guide in treatment decisions in inflammatory bowel disease. However, the process for stool sample collection is cumbersome and many patients strongly dislike it. Automated hands-free stool collection would increase adherence to regular monitoring. We have developed a Smart Sampling Toilet system that isolates feces downstream from a flush toilet without impacting the user experience.
Methods In the sampling toilet, a feces sample is collected by spray-erosion of isolated stool resulting in a fecal suspension in buffer of controllable solid content. A 15 ml fecal suspension in buffer of 30mg/ml solid content was used as Sampling Toilet specimen in this pilot study. Clinical stool specimens (n=25) were obtained from LabCorp with FCP values ranging from 50 and 500 μg/g. Each stool specimen was tested pairwise using standard sampling with an inoculum loop and using Sampling Toilet fecal suspension, after centrifugation at 10,000 g for 20 minutes (Figure 1). FCP in the standard and Sampling Toilet samples were measured simultaneously using colorimetric ELISA per manufacturer instructions.
Results The necessary amount of stool needed from the Smart Toilet sampling system for the ELISA assay was 100 mg. Smart Toilet suspension samples yielded more than adequate amounts of specimen for all the stools tested in study with a Bristol Scale ranging from 2 to 7. Similar calprotectin results were obtained using the standard vs novel Sampling Toilet approach (Figure 2). Selected clinical specimens were measured a second time in different experiments for a total of n=32 paired measurements. In three measurements, the FCP results exceeded the ELISA assay upper limit of 830 μg/g. Passing-Bablok linear regression (n=29 tests within the assay range) demonstrated that the two methods are statistically comparable (Figure 2A). Cusum test for linearity indicated no significant deviation from linearity (P > 0.05). A concordance matrix (n=32 tests) using cutoffs of 100 and 300 μg/g shows an agreement of 84% (Figure 2B).
Conclusion These data support the feasibility of automated stool sampling from the Sampling Toilet for FCP quantitative measurements. The Sampling Toilet platform holds potential to improve adherence to fecal tests and remote management of IBD and other chronic GI conditions.
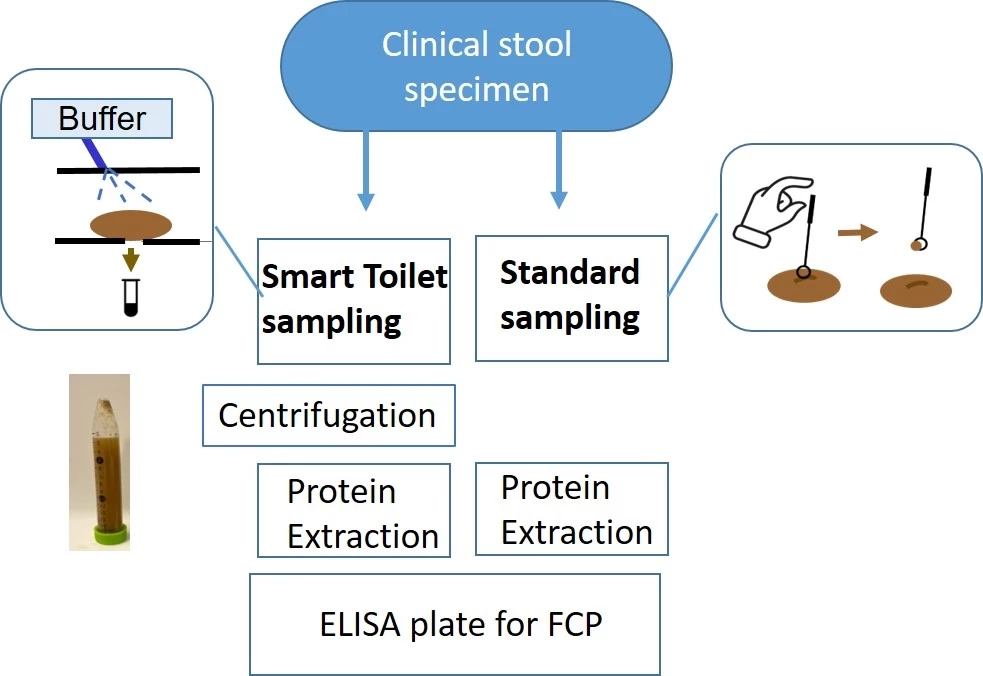
Figure 1. Study methodology. Fecal Calprotectin (FCP) matched pair-wise comparison between the two sampling methods (Sampling Toilet and Standard) was conducted on clinical stool specimens using ELISA.
Figure 2. Comparison of fecal calprotectin concentrations using standard and Smart Toilet sampling. Fecal Calprotectin (FCP) was measured by ELISA in the same stool specimen sampled by either the standard method or by the Smart Sampling Toilet. A Passing-Bablok linear regression of the two methods. Red thick line: the regression line; dotted line 95% confidence interval; solid line: the equality line. B Concordance matrix using 100 and 300 μg/g cutoffs shows 84% total agreement. TA=Target Agreement.